1 Studying the Earth
Learning Outcomes
By the end of this section you should be able to:
- Recognize, and differentiate between, hypotheses, theories, scientific laws, principles and models;
- Express very large and very small numbers in exponential format;
- Explain similarities and differences between observational and experimental scientific investigations;
- Recognize the use of principles such as uniformitarianism and actualism in Earth science;
- Identify branches of Earth science that utilize different types of observations.
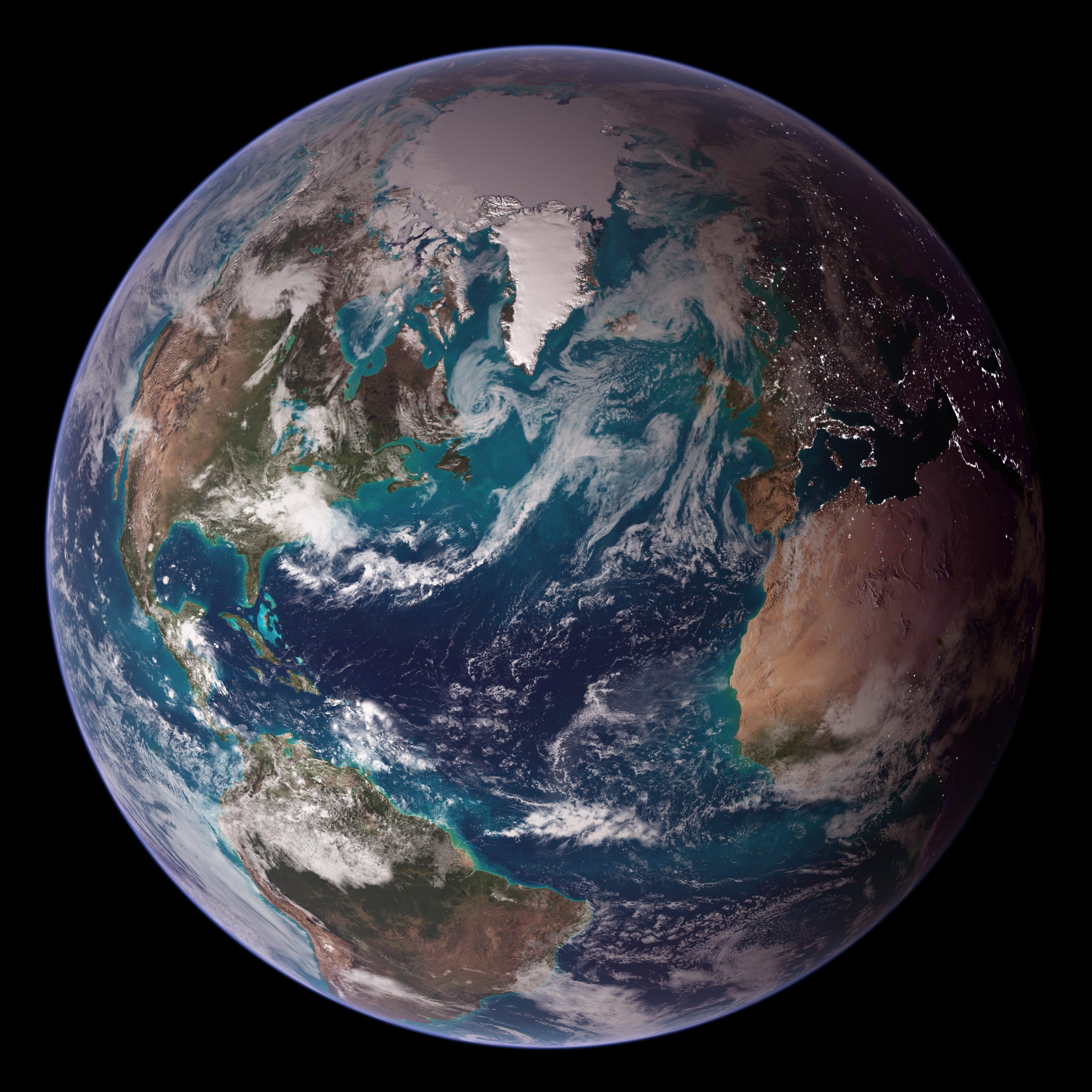
The Earth is a fundamental part of all our lives, and yet it’s very easy to forget about the Earth, and to make assumptions about how it works. The Earth seems solid and unchanging, as if it has always been the way it is now. Nonetheless, Earth scientists have shown that our planet is constantly changing, and that some of this change has been caused by Earth’s inhabitants, living plants and animals. In the most recent part of Earth history, humans have been responsible for huge changes. To understand the impact of these changes, we must understand what the Earth is made of and how it works.
In this first section we will look at the ways in which scientists study our planet, to find out how it has come to be the way it is, and how it continues to change. We will take a look at what makes the human activity we call science, how scientists accept and reject theories, and in particular, how Earth science differs from other sciences that you may be familiar with, such as biology, chemistry, physics, or astronomy.
What makes science?
Science is a branch of human activity that consciously aims at explaining the Universe around us. In some cases this is purely to satisfy human curiosity, but in other cases it leads to practical applications that we call technology: machines, new substances, sources of materials, food, water, and energy. There are many branches of science, but common to all of them are certain ways of working, involving observations, hypotheses and theories.
Observations

Fundamental to all sciences is the idea of observations: experiences that we describe in words, numbers, or diagrams. Earth science is predominantly an observational science (in contrast to experimental sciences like physics and chemistry). We typically make observations of features of our planet outdoors in natural environments. Unlike a laboratory bench, a natural environment is usually quite “messy”: we do not typically have the opportunity to set up a simple experiment in which only a few observations are possible. We simply do not have time to describe every tiny feature of our natural environment. Observations of natural environments are therefore somewhat selective, and the selection of observations is dependent on what hypotheses or theories we are interested in making or testing. Learning Earth science involves learning what observations are most important in different types of investigations. If you are doing a lab-based or field-based course on planet Earth, you will have the opportunity to make your own observations of Earth materials and natural environments. If your course is based only around lectures and this book, you should also look at the world around you, your own environment, and try to identify the features that we describe
Scientists develop systems of words to describe observations so as to make them more precise. In common speech different people may use different words to describe the same thing: what one person describes as blue, another may describe as green; we may avoid the problem by calling it teal. All sciences involve a certain amount of new vocabulary, typically designed to express observations precisely and with minimal ambiguity. The Earth sciences involve quite a lot of terminology, simply because the Earth contains many different things. In this course we try to keep the new vocabulary to the minimum necessary for the understanding of principles, and making good observations. New words are identified in the text and wherever possible defined in the glossary. If you go on to take higher-level courses about the Earth, you will meet many additional terms.
Some terms from Earth science have entered popular vocabulary too. We hear about “seismic” or "tectonic" shifts in opinions and the “epicentres” of human conflicts. It’s important to remember that these terms have more precise scientific definitions that may be different from their popular meaning. When you use words such as these in a scientific context, you should make sure that you are using them in the way they are meant by scientists.
*
Numbers: large and small
In using numbers, one challenge is that Earth science involves some numbers that are very small or very large. It’s important to ask “how small” or “how large” when observations are expressed in these terms. For very small and large numbers we typically use exponential format.
The number of seconds in a year is 31,556,926. We can write this in exponential format as:
3.1556926 x 107 s
or, approximating to three significant figures, as:
3.16 x 107 s
For very small numbers, the exponent is negative. For example the radius of an oxygen atom is about 0.00000000006 m or:
6 x 10-11 m
It’s also important to ask how precise a numerical observation is. If we say that two plates are moving past each other at 10 cm/yr, is that exactly 10 or could it be 9.9 or 10.3? In some cases the precision can be indicated by using an appropriate number of significant figures: ~10 (approximately 10) or just 10, or 10.0, or 10.00. For more detailed work it’s common to express precision with a plus or minus figure, for example
10.0 ± 0.3 cm/yr
For more help about numbers and units in Earth science, see the backgrounder on numbers and units.
Hypotheses
Much of science is based around the idea of a hypothesis: typically a statement of an idea about how observations are related to each other, and especially a statement of how observations can be explained. What are the processes that led to the observations?
This means that hypotheses are testable, and this is a fundamental feature of the scientific method. When we make a hypothesis to explain an observation, we hope that it will explain other observations in the future. To test hypotheses, physicists or chemists will usually set up an experiment, where they can control conditions inside some kind of apparatus, to see if the hypothesis correctly and repeatedly predicts the result. For astronomers studying distant galaxies, experiments are not usually possible. Instead, astronomers make repeated observations (for example of as many galaxies as possible) to see how consistently a hypothesis holds true. Biologists and Earth scientists are somewhere in between. Many of our observations are made “in the field”, outdoors in natural environments, but we can also collect samples and bring them to laboratories where they can be observed, analysed, and experimented on. For example, we may decide to heat rock or mineral samples, or subject them to mechanical forces. We do this to test our hypotheses about how similar materials formed in the natural world. One of the challenges for Earth scientists is that natural processes take place over huge spans of time: our laboratory experiments are limited by human lifetimes, so interpreting them in terms of what went on in the Earth can be difficult.
Theories and laws
A hypothesis that has survived many observational or experimental tests, and that explains more than a single phenomenon, is typically eventually described as a theory. Theories may explain anything from a part of the natural world to major features of the Universe.
Sometimes a well tested theory is broken down into one or more laws. A scientific law is a part of a theory that has survived rigorous testing, and can be stated in a simple sentence or two, or in a mathematical equation that relates numerical observations.

However, theories and laws are not unchangeable. New observations may lead to the modification or replacement of a theory with a new one. For example, in physics, Isaac Newton’s laws of motion were formulated in the 17th century and provided a basis for much of physics until the early 20th century. However, at that time, it became possible to measure the speed of very fast-moving particles, and even to measure the speed of light. It was discovered that while Newton’s laws of motion worked well for observations of objects travelling at human speeds, they failed to predict observations made of these high-speed particles and the behaviour of light itself.

Einstein’s theory of relativity was developed to explain these observations in the 20th century and has largely replaced Newton’s laws in the fundamental understanding of the underlying processes. However, it’s important to understand that Newton was not really wrong. When we use Einstein’s theories to predict the behaviour of particles that are moving at speeds typical of human experience, the predictions are not measurably different from Newton’s. We say that Newton’s simpler theory is an “end-member” of Einstein’s relativity: it works perfectly well for phenomena that occur at the speeds of everyday objects.
An example from Earth science is the theory of continental drift. This theory was developed early in the 20th century to explain similarities between the continents on either side of the Atlantic ocean. It proposed that the continents moved, a bit like large floating icebergs, through the material of the ocean floors and the Earth’s mantle below. The theory of continental drift was vigorously opposed by many Earth scientists in the mid-20th century because it appeared to require properties of the Earth’s ocean floors that were in conflict with observations about how materials could behave.

In the late 20th century, the theory of plate tectonics was developed. Plate tectonics resolved most of the problems of the earlier concepts of continental drift, by proposing that both the continents and the ocean floors moved together as rigid plates, and that movement occurred along narrow plate boundaries. Plate tectonics was thus able to explain all the same observations as continental drift, but also explained many more observations (for example, observations of the ocean floor, earthquakes, and volcanoes) that were not addressed in the earlier theory. Plate tectonics has become almost universally accepted by Earth scientists. Most recently (in the present century) it has become possible to measure the movement of the plates using GPS (global positioning system) technology, providing a further test of its predictions.

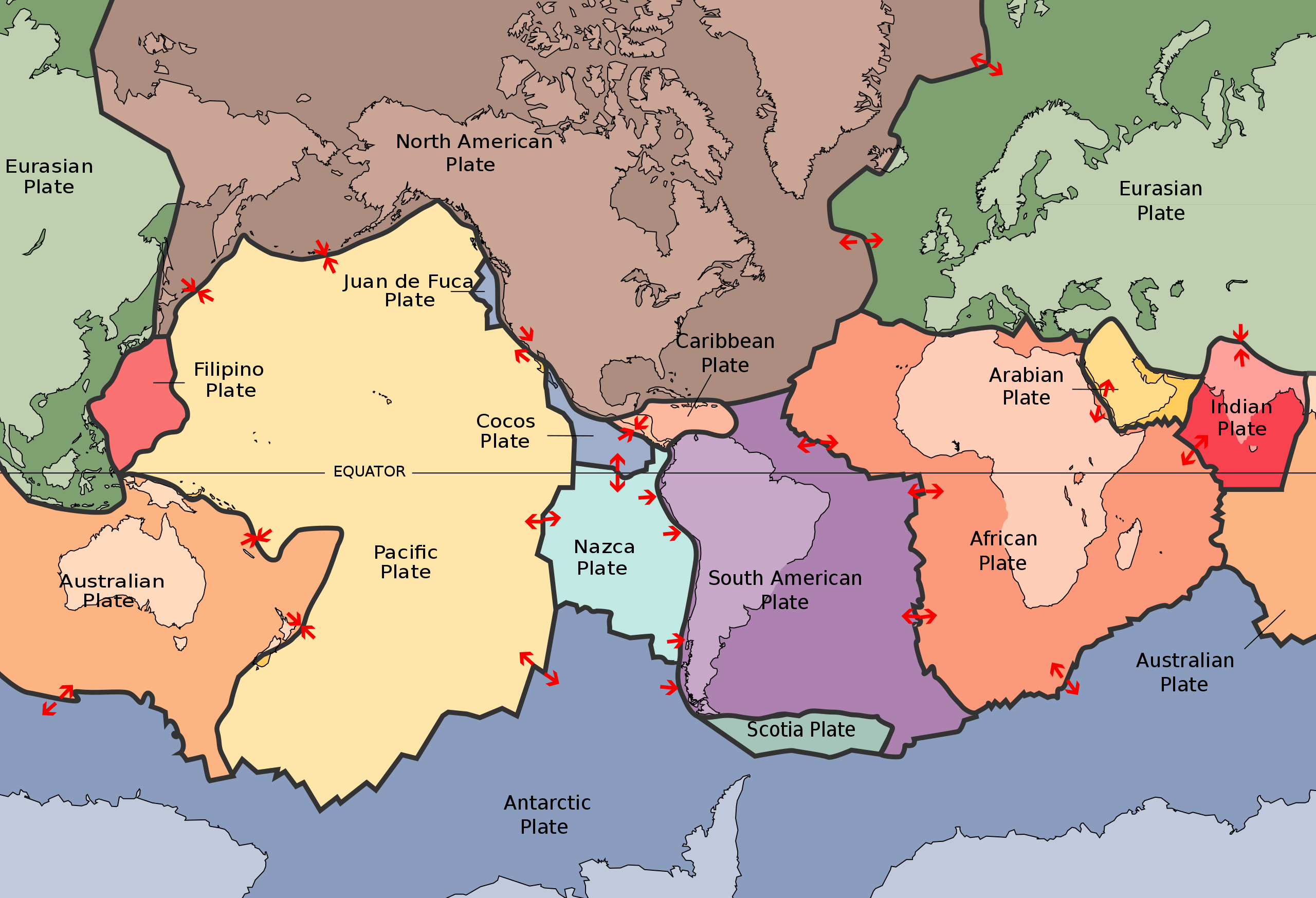
Principles
Sometimes a hypothesis is useful despite being shown to have exceptions. An example from Earth science is the “principle of superposition”. This states that when we observe layers of rock, the upper layers are younger than the lower ones (because they were deposited later). Thus rule has even been called the “law of superposition” but most Earth scientists do not call it a law because there are many exceptions. For example, a layer of molten rock may work its way along a crack between layers that were deposited millions of years previously, and solidify to form a structure called a sill. In other examples, we find places where movement of plates has tilted the original layers and even turned them upside down.

The principle is still useful because it works most of the time, and we have learned how to make observations of the clues that tell us when it’s not working. For example, molten rock that forms a sill typically bakes the rock above and below, and we have learned (through observation and experiment) to recognize rocks that have been baked.
Rules like this are known as principles when they are useful in explaining things and making new hypotheses, but are not universally found to be true.
Models
Because the natural world is an exceedingly complex place, observations in Earth science are much more difficult to interpret than observations made in a laboratory-bench experiment designed by chemists or physicists. In such experiments, it’s often possible to change just one variable at a time (for example the temperature or the pressure within the apparatus) to see what is the effect on the observed results.
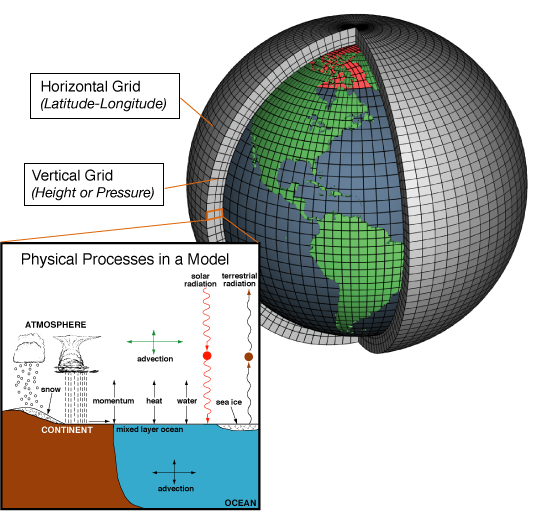
In Earth science, nature effectively carries out our experiments for us and we don’t have the ability to change just one variable at a time. It’s common therefore, to set up "models" – simplifications of the natural world in which we can control the input variables. The word model covers an astonishing variety of scientific constructions. A model may be an actual physical construction on a laboratory bench that simulates an Earth process in simplified form, or it may be a set of mathematical equations, or algorithms, that are made to run in a computer simulation. For example, weather forecasters increasingly rely on general circulation models, or global climate models, that are continuously running on major computer installations. The same models that are used to predict weather may be used to predict the effects of human changes to the atmosphere. These models can often be tested, once time has elapsed, by comparing their results with new observations.
Paradigms

The theory of plate tectonics is sometimes called a paradigm (pronounced “paradime”). What does this mean? The term paradigm was introduced by the influential philosopher of science, Thomas Kuhn, in the 1960s. Kuhn investigated the way major new scientific theories, theories that revolutionized a certain field, were closely related to other events in society, and suggested that the motivations for these scientific revolutions were not just the failure of previous theories to correctly predict observations, but were closely related to changes in scientists’ views on just what observations needed or could to be interpreted. The combination of a new theory with new ideas about what could and should be explained was called a paradigm in Kuhn’s explanation of how science works as a human enterprise.
Kuhn’s book, “The Structure of Scientific Revolutions”, looked at major revolutions that had occurred in physics (Newton’s mechanics, and then Einstein’s relativity) and biology (Darwin’s theory of evolution) over the previous centuries. However, at the same time that Kuhn was writing, Earth science was undergoing its own revolution, the discovery of plate tectonics. Plate tectonics fits Kuhn’s idea of a scientific revolution very well. During the 1960s, there was a radical change in most Earth scientists’ view of the Earth, from one in which the continents and oceans were largely fixed, to one where the outer shell (or lithosphere) of the Earth was divided into moving plates. Some of the evidence for plate tectonics came from outside the previous community of Earth scientists. For example, as a result of international treaties to limit the testing of nuclear weapons, it became imperative to detect atomic explosions and differentiate them from earthquakes. This led to the setting up of a worldwide synchronized network of seismometers for monitoring compliance with the treaties. An incidental spin-off from this new data source was hugely improved information about the timing of earthquakes, their locations, and the movements that caused them. New maps of earthquake epicentres made plate boundaries much more obvious than before. Plate tectonics thus caused Earth scientists to consider a host of new types of observations, and to alter their perceptions of what could be achieved by the science. It thus fits Kuhn’s definition of a paradigm remarkably well.
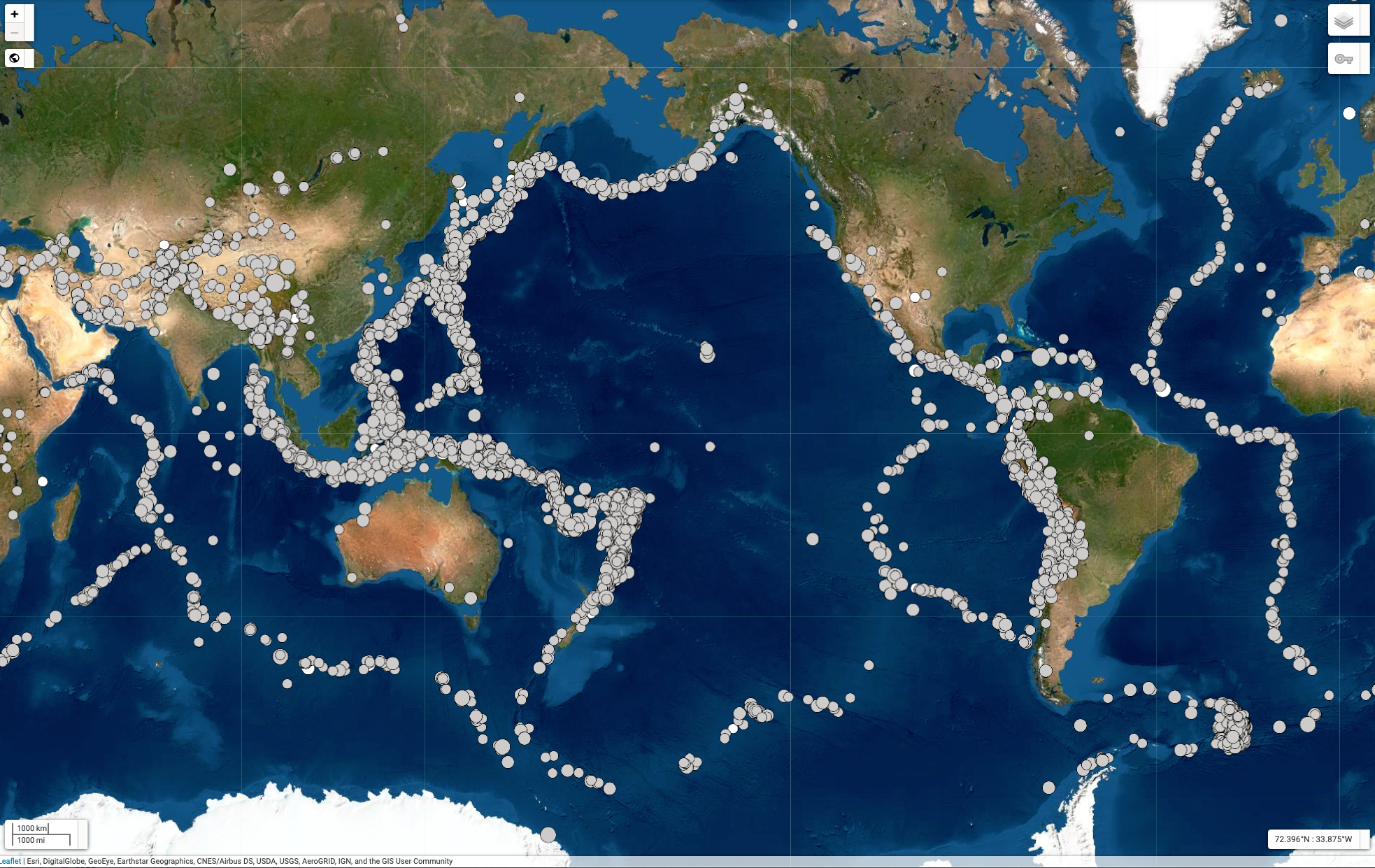
What’s special about Earth science?
Earth science has some distinctive features that distinguish it from other sciences. Many descriptions of science emphasize experiments, but in most areas of Earth science, laboratory experimentation is less important than observations made in natural environments. Earth science also has to deal with enormous lengths of time. This section discusses these special features of Earth science.
Observation vs experiment
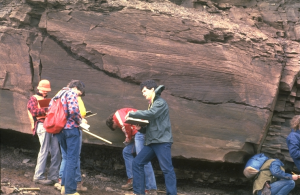
Many discussions of the scientific method emphasize experiments. Experiments are the major means of hypothesis testing in physics and chemistry, but are less important in the Earth sciences, because it’s practically not feasible to reproduce a complex, and possibly very large and long-lasting Earth system on a laboratory bench. Most branches of Earth science are therefore founded on fieldwork, the collection of observations in a natural, outdoor environment. Fieldwork in Earth science ranges from very simple (for example, hiking outdoors recording observations on a map) to extremely complex (sending remote-controlled aircraft into the stratosphere, or generating seismic waves that penetrate deep into the Earth before being recorded as they bounce back from hidden layers to the surface).
Fieldwork may involve collecting samples of Earth materials: atmospheric gases, types of water, or samples of rocks from the solid Earth. Often samples are returned to a laboratory for analysis, to discover more about them than can be discerned in the field. The analysis of Earth materials has become extremely sophisticated; the design of analytical instruments requires knowledge of of principles of physics or chemistry, as well as technological expertise in the construction of the instruments themselves. The interpretation of the results is typically dependent on these other sciences.
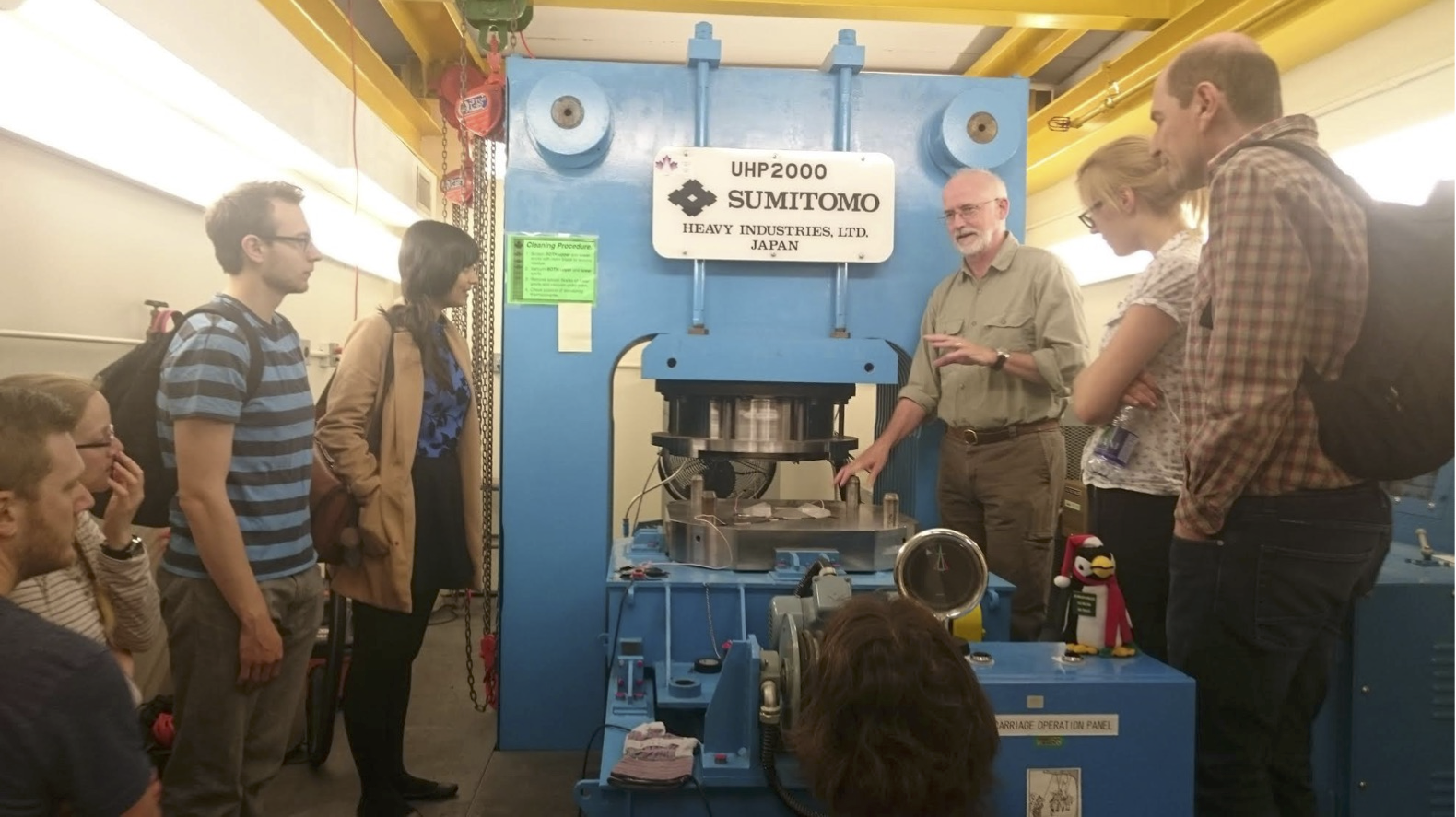
In some cases, Earth scientists do also carry out experiments. For example, they may heat natural samples of rock, or artificially generated samples of known composition, to determine how they behave under great heat or pressure. The resulting observations may be compared with those made from samples of natural rocks, to help estimate the pressures and temperatures that those rocks have been subjected to.
The time dimension
Experimental work in Earth science is limited by some important constraints. First, processes within the solid Earth operate extremely slowly, at least when considered on human time scales. Geologists – Earth scientists who focus on the materials and history of the Geosphere – become accustomed to talking about lengths of time measured in millions and billions of years. In contrast, it’s extremely difficult and expensive to run an experiment for periods of longer than a few months or years. Therefore geologists who carry out experiments to investigate Earth processes must either use analog materials (soft materials that react faster than natural rocks) or they must extrapolate from a short-duration experiment to predict effects that occur over much longer time scales. Neither of these strategies is particularly easy!
The present as a key to the past: Uniformitarianism and actualism

One useful strategy in dealing with the limited time-scale of observations is using the principles and laws developed in other sciences that investigate the present-day world, particularly physics, chemistry, and biology. This idea was proposed in the 18th century by Scottish natural philosopher James Hutton. Hutton formulated a principle known as uniformitarianism. This principle holds that processes that operated in the Earth’s past can all be explained by processes that continue to operate at the present day. This principle was very radical when first proposed: most other natural philosophers believed that the history of the Earth involved creation events followed by great catastrophes, after which very little was the same.
Our modern understanding is more flexible than Hutton’s. Although reference to present-day processes is extremely useful in interpreting the past, it is clear that observations of the older parts of the Earth cannot all be explained by processes identical to those going on at the present day. For example, it’s clear that for about half of its history, Earth’s atmosphere contained little or no oxygen. The earliest sedimentary rocks were therefore deposited in environments that were not exactly the same as any that exist at the present day. Another hypothesis that Hutton would have rejected as contrary to uniformitarianism is the idea that the dinosaurs were wiped out by an asteroid impact about 65 million years ago, because such a process has not been observed in human lifetimes. Despite the unfamiliarity of these processes to modern humans, it is clear that they were governed by physical and chemical laws identical to those operating today, and can be interpreted using those laws. This more modern perspective on the past is known as actualism – the principle that events in the past can be explained in terms of physical, chemical, or biological processes and laws that operate at the present day. Succinctly stated as “the present is the key to the past”, the principle of actualism lies at the heart of many aspects of modern Earth science.
Branches of Earth science: relationship to other sciences
By now it should be clear that Earth science overlaps with many other scientific disciplines, and these overlaps define branches of Earth science that have their own names. Very few Earth scientists are able to keep on top of developments across the whole field. Most will characterize their activities as falling into a sub-discipline like atmospheric science, oceanography, paleontology, geology, or geophysics:

- Atmospheric scientists focus on the Earth’s gaseous atmosphere. Amongst atmospheric scientists, meteorologists focus on day to day changes in weather whereas climatologists investigate longer-term changes in the atmosphere.
- Oceanographers study the Earth’s seas and oceans, whereas hydrologists study water on the land surface. A closely related subdivision, hydrogeology, deals with water within the pore spaces in the outer parts of the solid Earth, the geosphere. Glaciologists study water in its solid form, ice.
- Geologists study the geosphere, the largely solid Earth, with emphasis on its materials (rocks and minerals) and the history recorded by the materials that can be seen near the Earth’s surface. Geophysicists also study the geosphere, but using indirect methods of observation based on its physical properties such as the Earth’s magnetism, the behaviour of seismic waves, the flow of heat through and from the Earth, and the electrical behaviour of the Earth’s interior. Much of our knowledge of the deep interior of the Earth has been obtained through geophysics.
- Paleobiologists study ancient life forms, principally through observations of fossils; paleontology concerns animal fossils whereas paleobotany concerns plants. Often, these ancient life forms are interpreted by comparison with the distribution and interactions between modern life-forms, studies known as biogeography and ecology.
Our journey through the spheres of the Earth will involve visits to all these enormously diverse fields. Each of them has involved lifetimes of scientific work. This course focusses on the broad interactions between these fields of Earth science. If you find one that you are particularly interested in, you may wish to take more advanced, and therefore more specialized courses, to further your knowledge and pursue your interests. In this course we will keep our introduction to the Earth as broad as possible.
The third planet from the Sun in the Solar System, and the principal object of study for geoscientists; the only planet known to possess life.
Relating to movements of the Earth's lithosphere
A point on the Earth's surface located vertically above the focus, or hypocentre, of an earthquake
A way of representing large or small numbers using a coefficient or mantissa (typically a number with one digit to the left of the decimal point) multiplied by an exponent (a power of 10)
An statement that explains or predicts observations, and is therefore testable
A hypothesis, or group of hypotheses, that has survived many experimental or observational tests, leading to general acceptance of its explanatory power
A theory or part of a theory that has withstood repeated testing and can be succinctly stated in words or as a mathematical equation.
A group of theories in physics that state that the laws of mechanics and the speed of light are the same for all observers, even if those observers are moving relative to each other
A theory that the Earth has a lithosphere that is divided into relatively rigid moving plates that interact along plate boundaries
A simplified or theoretical version of a complex natural system, either a physical model using analog materials, or a mathematical model run on a computer.
Computer-based models of how the atmosphere behaves. Although these models can be very complex, they represent simplified versions of the real atmosphere. Global climate models are typically types of general circulation model. The same abbreviation (GCM) applies to both.
A new collection of objectives, methods, and theories for a scientific discipline, developed during a scientific revolution
Breaking down something into its component parts
The solid Earth
The principle that processes that operated in the Earth's past can all be explained by processes that continue to operate at the present day. Strict application of uniformitarianism in Earth science has largely been replaced by actualism
The principle that events in the past can be explained in terms of physical, chemical, or biological processes and laws that operate at the present day
The study of the atmosphere of a planet (usually Earth).
Atmospheric scientists who study short term changes in the atmosphere known as weather.
An atmospheric scientist who studies long-term changes in the Atmosphere
The study of Earth's seas and oceans
A scientist who studies the flow of water at the Earth's surface
The study of water within the Geosphere
A scientist who studies glaciers and ice in the Earth system
A scientist who studies the Geosphere using on observations of Earth materials
A scientists who studies the interior of the Earth mainly through remote sensing methods using physical properties of the Earth.
A scientist who studies the remains, lives, and evolution of ancient living things
The study of fossil animals; often used to describe the study of all fossils, including plants, where “paleobiology” would be more strictly correct.
The study of relationships between groups of organisms interacting with their physical environment